Quantum error correction with silicon spin qubits
Silicon-based spin qubits offer an outstanding nanofabrication capability for scaling up. Here we demonstrate a basic quantum error correction using silicon spin qubits. We implement a Toffoli-class three-qubit gate by a single pulse under simultaneous exchange coupling. We then synthesize a three-qubit repetition code to demonstrate the correction of a phase flip error on one of the three qubits.
We accurately control the three-spin system defined in a triple quantum dot of silicon/silicon-germanium by electric-dipole spin resonance and adiabatic pulsing of exchange coupling. We combine the single-qubit and two-qubit gates to encode a spin qubit state to a three-qubit entangled state. Then we implement a three-qubit Toffoli gate by a single rotation pulse under simultaneous nearest-neighbor exchange interaction.
We intentonally introduce a phase error with various error angles in one of the three qubits and measure the process fidelity of the data qubit with and without error correction. The measured fidelity decreases monotoniclly with increasing error angle withou error correciton,whereas well recovered with error correction, indicating the errror correciton protocol works well.
This is the first proof-of-principle experiment of error correciton in the spin qubit system, and will encourage development of scalable multi-qubit devices in silicon.
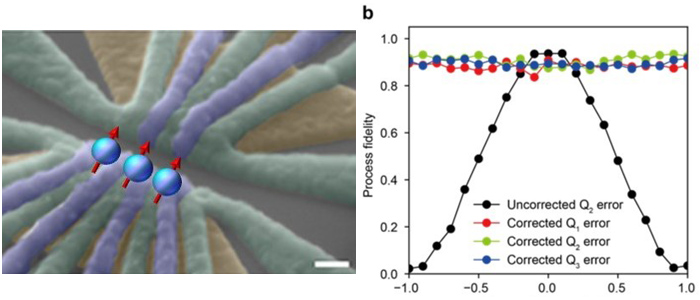
Figure (Left) Silicon three quantum bit device used for the experiment. (Right) Result of quantum phase error correction (Error recovery for the data qubit Q2).
High-fidelity universal quantum gate set using electron spin qubits in silicon
One of the conditions that a fault-tolerant quantum computer requires to satisfy is fidelity of universal quantum gate set above 99%. In silicon spin qubits, the single-qubit gate fidelity exceeding 99.9% was demonstrated. In contrast, the two-qubit gate fidelity was limited to 98% due to a slow operation compared to spin dephasing. Here we overcome this problem and achieve the two-qubit gate fidelity of 99.5% by making faster the gate speed by an order of magnitude than before.
We fabricated a silicon quantum dot device in an isotopically enriched silicon quantum well/silicon germanium heterostructure with a micromagnet on top, which enables high-speed single-qubit rotation by an electric-dipole spin resonance under a magnetic field.
We used the fast spin rotation and a large exchange coupling to realize a fast CNOT gate, the most important two-qubit gates, and achieved the single-qubit gate fidelity of 99.8% and the two-qubit gate fidelity of 99.5%.
The result shows that silicon spin qubits can compete with the most advanced qubit systems of superconductor qubits and ion trap qubits.

Figure (Left) Silicon quantum bit device used for the experiment. (Right) Characterization of the two qubit gate fidelity using randomized benchmark method.
Quantum non-demolition measurement of semiconductor quantum bits
Quantum computing with single electron spins in silicon has been intensively studied,motivated by prospect for the qubit scale-up using semiconductor device processing technology. However, implementing useful measurement-based protocols, including error correction remains a challenge because the qubit measurement usually demolishes the spin state. Here we probe a neighboring electron spin Ising-coupled to the qubit spin and first succeed in the quantum non-demolition measurement of the electron spin in silicon.
In this experiment an electron spin is first initialized to an arbitral qubit state (main qubit) using a spin resonance technique. Next, an Ising-type coupling is applied to the main qubit and the partner qubit (ancilla qubit) to form entanglement between them. When the ancilla qubit is measured being spin-up or spin-down, the main qubit is readout without measuring it. This is a nondemolition projective measurement that causes no substantial error in the main qubit, and allows to raise the readout fidelity of the main qubit by repeating the ancilla measurement.
Our work offers a promising route to construct an error correction circuit in silicon.
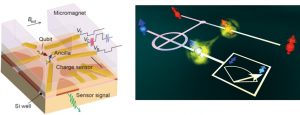
Figure (Left) Schematic view of the quantum double dot device used for the experiment. (Right) Concept of quantum nondemolition measurement.
An ultra-high fidelity quantum dot qubit in a silicon quantum dot
The building block of quantum computers, or the smallest unit of quantum information, is called a qubit. A very large number of high-quality qubits will be needed to build a quantum computer. Single electron spins in quantum dots are a strong candidate as qubits, as they likely benefit from modern electronics integration technology, once sufficient quality is reached.
Enhancing the qubit quality means improving both coherence time and control time, challenging the trade-off commonly observed between these. In this work, we fabricated a quantum dot on an isotopically-clean silicon wafer to increase the coherence time, and deposited a micromagnet nearby to decrease the control time by its slanting magnetic field. With the coherence time ten times longer and the control time two orders of magnitude shorter than conventional within a single device, we implement highly coherent qubit operations (Fig. B). We further demonstrate >99.9% control fidelity (precision), above the fault-tolerance threshold.
Our work offers a promising route to large-scale, ultra-high-fidelity spin-qubit systems in silicon.

(Fig. A) Schematic view of the quantum-dot qubit device
(Fig. B) Chevron resulting from ultra-high fidelity spin rotations