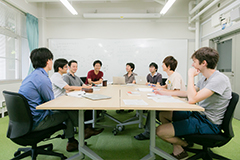
By means of first-principles methods, our team studies non-trivial electronic properties of materials which lead to new ideas/notions in condensed matter physics or those which have potential possibilities as unique functional materials. Especially, we are currently interested in strongly correlated/topological materials such as high Tc cuprates, iron-based superconductors, organic superconductors, carbon-based superconductors, 5d transition metal compounds, heavy fermions, giant Rashba systems, topological insulators, zeolites, and so on. We aim at predicting unexpected phenomena originating from many-body correlations and establishing new guiding principles for materials design. We are also interested in the development of new methods for ab initio electronic structure calculation.
Classification of emergent responses of magnetic materials based on spin crystallographic groups
The spin, an intrinsic degree of freedom of electrons, brings about diverse physical properties through its ordering (magnetism). In particular, when there is the spin-orbit interaction (SOI), which links the spin and orbital degrees of freedom, magnetism is associated with the physical properties of materials, such as electrical conductivity and elasticity. This is evident in phenomena like the anomalous Hall effect and magnetostriction. However, the impact of SOI is limited in elements such as Fe and Mn due to its relativistic nature, which constrains material exploration. On the other hand, thanks to their non-trivial magnetic structures, some antiferromagnets show emergent electromagnetic responses independent of SOI.
When exploring the properties of such antiferromagnetic materials, analyzing the symmetry of magnetic structures plays a crucial role. Magnetic space groups are commonly used for this purpose, but they implicitly assume the presence of spin-orbit interaction, making them unsuitable for the symmetry analysis of emergent electromagnetic phenomena. We have focused on spin space groups that do not consider the constraints of spin-orbit interaction. We developed a method to conveniently identify magnetism-driven properties. This method serves as a guideline for understanding and predicting emergent properties.

Example of a non-coplanar spin structure with nontrivial spin space group symmetry.
Ab initio prediction of magnetic structures
In a magnetic material, some of the atoms in a crystal carry magnetic moments, which align themselves with their neighbors in very specific formations. The smallest building block of a magnetic material -the magnetic structure- fundamentally determines the properties of a magnet. Yet the prediction is highly nontrivial due to the many degrees of freedom in the system. We formulated a scheme to predict the magnetic structure from first principles by combining the cluster multipole method and spin density functional theory, in which we start with a sophisticated list of possible spin configurations.
The list of configurations is found in a spherical expansion analogous to how atomic orbitals are constructed, but highly adapted to the crystal symmetry. We then performed a high-throughput calculation with close to 3,000 possible magnetic structures for 131 materials and found many stable and meta-stable magnetic structures. We compared our prediction to the reference standard, which we aim to reproduce, that is, the experiment. This kind of high-throughput benchmark calculation showed that our approach can significantly narrow down the possible properties of a material.
With this scheme, the exploration of magnetic materials is pushed to enter a new paradigm of material design that lives up to the era of big data.

Scheme for magnetic structure prediction.
Quantum crystal structure in the 250-kelvin superconducting lanthanum hydride
Condensed-matter physicists have devoted enormous efforts to finding a room-temperature superconductor. Recently, it has been reported that LaH10 with a hydrogen cage enclosing the lanthanum atoms is formed at pressures higher than 130 GPa, and becomes a superconductor at a temperature between 250 and 260 K—about 40 K below room temperature. According to conventional (classical) calculation based on the Born-Oppenheimer approximation, the highly symmetric cage structure which favors superconductivity is stable only for pressures higher than 230 GPa, and it would distort at lower pressures.
We performed a calculation where atoms are treated like quantum objects, which are described with a delocalized wave function. While the energy landscape in the classical calculation is very complex with many minima, it is entirely reshaped by considering the quantum effect: There is only one minimum, which corresponds to the symmetric structure. We also performed a first-principles Eliashberg calculation and succeeded in reproducing the pressure dependence of the superconducting transition temperature.

Crystal structure of LaH10, together with a sketch of the complex classical energy landscape (top) and that of the reshaped quantum energy landscape having only one minimum (bottom).
Cluster multipole theory for functional antiferromagnets
Recently, functional antiferromagnets are attracting an increasing amount of attention. In contrast with ferromagnetic devices, those using antiferromagnets have several advantages: They are robust against magnetic field perturbations, which is good for data retention. Since they do not have strong stray fields, one can integrate high-density memory. The energy scale of antiferromagnets is usually higher than that of ferromagnets, which is convenient for ultrafast data processing.
Motivated by the discovery of an extremely large anomalous Hall effect (AHE), anomalous Nernst effect (ANE), and magneto-optical Kerr effect (MOKE) in Mn3X (X=Sn, Ge), we introduced a new order parameter, which we call cluster multipole (CMP) moment, and showed that CMP characterizes AHE/ANE/MOKE in antiferromagnets with general spin configurations. We also found that CMP is useful for constructing database for magnetic structure and designing new functional antiferromagnets.
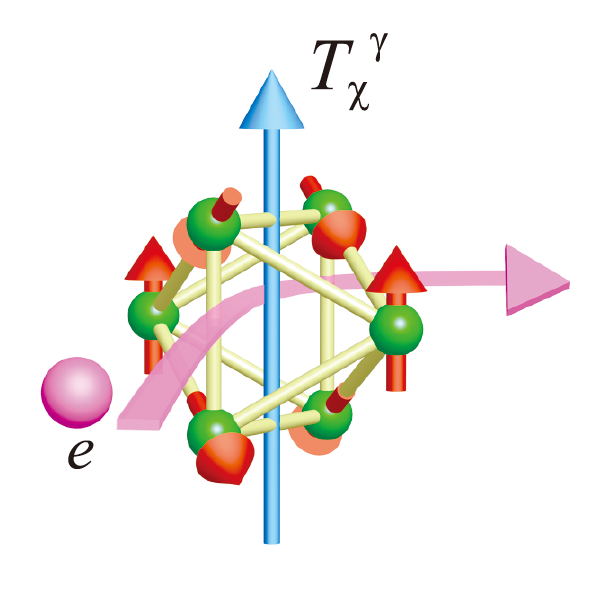
Cluster octupole in Mn3X (X=Sn, Ge), which induces a large anomalous Hall effect, anomalous Nernst effect and magneto-optical Kerr effect.